Pulsating cardiomyocyte bridge spanning a pair of micro-pier structures on a sheet of PDMS. The cultured cells spontaneously form bridges across the piers. Muscle contraction moves the flexible piers, which act to pump fluid.
Microdevices—a seemingly simple concept, but actually quite complex. Not to be confused with microchips that power your computer or phone, microdevices play an important role in the field of biomedicine. Many types of microdevices contain miniaturized biochemical systems in which spatial and temporal control of cell growth and stimulating factors are made possible by combining surfaces that mimic natural structures with channels that regulate the transportation of fluids and soluble factors. For example, scientists and engineers have been developing pulmonary and cardiac systems on microchips—often referred to as organs-on-chips—as a means to study these systems, replicate pathologies, and test treatments.
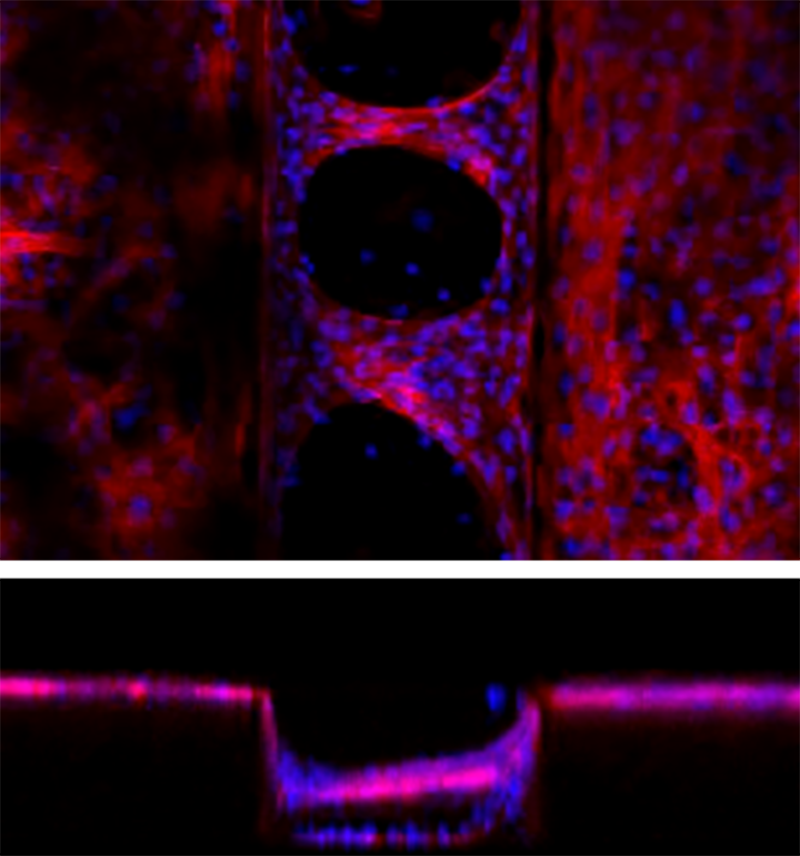
Confocal microscopy of cardiomyocyte bridging on a PDMS microgroove structure. Groove width is 200 μm and groove depth is 150 μm.
An essential component of this type of device is the micropump. Currently, microdevice applications are limited because these pumps are typically powered by external energy sources like batteries, making it difficult to really miniaturize them down to the “micro” level. However, a recent publication by an international joint research team led by Nobuyuki Tanaka and Yo Tanaka at the RIKEN BDR reported the fabrication of the world’s smallest autonomous hybrid pump. This micropump, made from a silicon-based organic polymer called polydimethylsiloxane (PDMS), is powered by cardiomyocytes (heart muscle cells) that self-organize into muscle tissue forming a cardiomyocyte bridge on the elastic PDMS microstructure.
How did they do it?
The team at BDR cultured rat cardiomyocytes on PDMS sheets with micro-grooves. They found that the cells spontaneously formed a three-dimensional pulsatile tissue that bridged the gaps between the grooves. Experiments showed that the highest number of bridges formed when the distance between grooves was 200 micrometers (0.2 mm). This is important because more bridges means more force is generated when the tissue contracts.
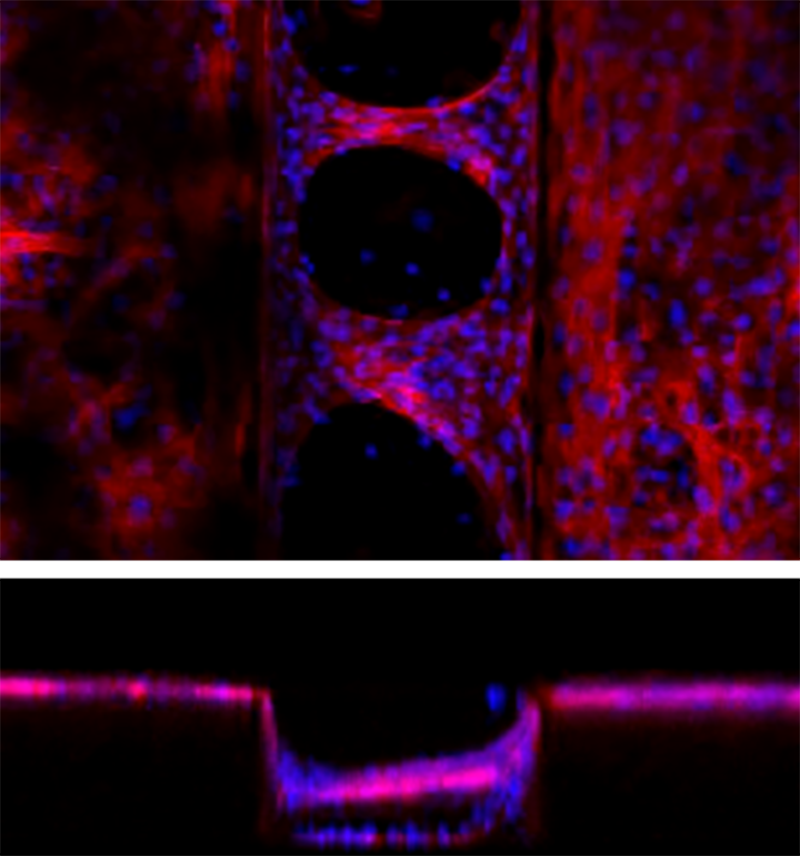
Confocal microscopy of cardiomyocyte bridging on a PDMS microgroove structure. Groove width is 200 μm and groove depth is 150 μm.
An essential component of this type of device is the micropump. Currently, microdevice applications are limited because these pumps are typically powered by external energy sources like batteries, making it difficult to really miniaturize them down to the “micro” level. However, a recent publication by an international joint research team led by Nobuyuki Tanaka and Yo Tanaka at the RIKEN BDR reported the fabrication of the world’s smallest autonomous hybrid pump. This micropump, made from a silicon-based organic polymer called polydimethylsiloxane (PDMS), is powered by cardiomyocytes (muscle cells from the heart) that self-organize into muscle tissue before forming a cardiomyocyte bridge on the elastic PDMS microstructure.
How did they do it?
The team at BDR cultured rat cardiomyocytes on PDMS sheets with micro-grooves. They found that the cells spontaneously formed a three-dimensional pulsatile tissue that bridged the gaps between the grooves. Experiments showed that the highest number of bridges formed when the distance between grooves was 200 micrometers (0.2 mm). This is important because more bridges means more force is generated when the tissue contracts.
When we saw the cultivated cardiomyocytes spanning across the grooves, we were really surprised because this formation is completely different from what happens without a grooved microstructure. Hundreds of cells gathered and aligned themselves to cross the grooves and the tissue seemed like real muscle tissue. In cell biology, the configuration of materials that are in contact with cells is known to strongly affect their function. Therefore, we believe this is quite an important achievement that can be a steppingstone for understanding how to generate tissues and organs with appropriate shapes and functions, how to artificially fabricate functional cellular tissue, and how to incorporate biological tissue into mechanical systems.
When we saw the cultivated cardiomyocytes spanning across the grooves, we were really surprised because this formation is completely different from what happens without a grooved microstructure. Hundreds of cells gathered and aligned themselves to cross the grooves and the tissue seemed like real muscle tissue. In cell biology, the configuration of materials that are in contact with cells is known to strongly affect their function. Therefore, we believe this is quite an important achievement that can be a steppingstone for understanding how to generate tissues and organs with appropriate shapes and functions, how to artificially fabricate functional cellular tissue, and how to incorporate biological tissue into mechanical systems.
After determining the best groove configuration, the team built a device that could use the pulsating bridges as an actuator to pump fluid. When the cardiomyocyte bridges formed between two tiny elastic pillars (50 micrometers in width, 150 micrometers in height) that were surrounded by fluid, the pillars bent inward when the bridges contracted. This pushed the fluid—and voilà, an autonomous micropump was born.
These research results have the potential to become the basis for experimental models of the cardiac system, or as a system for studying cardiotoxicity and testing the efficacy of drugs. In this study, the team used cardiomyocytes from rats. However, the ultimate goal is to generate cardiomyocyte bridges from induced pluripotent stem cells (iPS cells), which will allow the development of an experimental platform that reduces the reliance on clinical trials in the study heart disease. In the future, if it can be combined with a fine valve-like structure, the team expects to be able to create a “micro-heart”, a very small pump that is driven solely by biological energy sources. In fact, the team has already had success creating a muscle-driven microvalve. Further study of the cardiomyocyte-bridge phenomenon is expected to contribute to basic research into the development and functional expression of the heart and muscle.
A first draft of this article was written by Emily Bian, a RIKEN BDR intern from the University of Wisconsin, USA. ✅
Further reading
Tanaka N, et al. (2019) An ultra-small fluid oscillation unit for pumping driven by self-organized three-dimensional bridging of pulsatile cardiomyocytes on elastic micro-piers. Sensors Actuators B Chem, 293:256–264. doi:10.1016/j.snb.2019.04.087.