Welcome back to the It Ain’t Magic science blog. Before the winter break and the announcement about the discovery of element 113, we were talking about electroecosystems at [itg-tooltip tooltip-content=”<p><strong>Black smokers</strong> are a type of deep sea hydrothermal vent located in the ocean. They support diverse ecosystems in which the primary producers in the food chain (mostly bacteria) do not use sunlight for their energy.<a href=”]black smokers[/itg-tooltip], and I promised to tell you about a bacteria that can use either inorganic material or direct electricity as an energy source.
As you might remember, the discovery that electric current flows from the inside of a black smoker chimney to the outer surface led scientists to hypothesize that some microorganisms called [itg-tooltip tooltip-content=”<p><strong>Chemolithoautotrophs</strong> are microorganisms like those living near the black smokers that use chemical energy to make their own fuel from inorganic material.</p>”]chemolithoautotrophs[/itg-tooltip] might be able to double as electrolithoautotrophs, using electric current as a source of energy. The short version of the story is in a collaborative effort, members of the same team, led by Ryuhei Nakamura (now at the RIKEN Center for Sustainable Resource Science) experimented with the bacteria Acidithiobacillus ferrooxidans, and found that even when iron was absent, it could directly use electric potential to fuel growth. They can literally run on batteries. But how …?
Some background …
First, let’s begin with what we know about chemolithoautotrophs. These guys can synthesize the organic molecules they need to survive by using chemical energy gained from reactions with inorganic sources. For example, A. ferrooxidans is a bacterium that can remove electrons from ferrous iron (Fe2+ ions) and use them to eventually fuel sugar production.
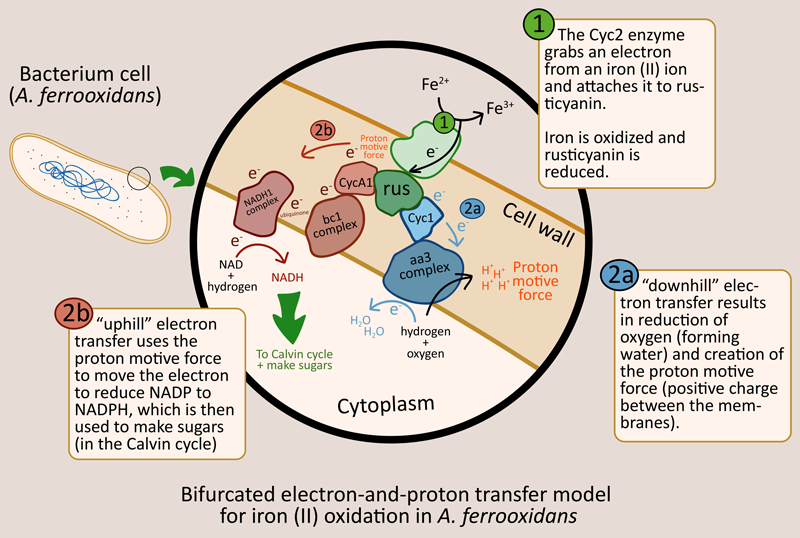
Iron oxidation yields electrons that are passed along, eventually used in carbon fixation to make sugars
[Beware: really interesting details follow] Electrons are first grabbed by the protein rusticyanin with the help of an enzyme called [itg-tooltip tooltip-content=”<p>aka <strong>iron-rusticyanin reductase</strong>, because it reduces rusticyanin (rus) by adding the stolen (from iron) electron to it. In the diagram above, Cyc2 is the green blob without a name.</p>”]Cyc2[/itg-tooltip]. From there, there are two possible pathways the electron can follow (see figure below, it has pretty colors and arrows and stuff). The first pathway is called the “downhill” pathway because it does not require any extra force. Electrons are passed along until they reach an complex that hands them off to oxygen ions, in the process pumping hydrogen ions (H+) from the cytoplasm to the space between the two membranes.
The extra H+ creates a difference in charge across the membrane (increasing the [itg-glossary glossary-id=”2257″]electric potential.[/itg-glossary] This is just like how positive and negative charges are separated to the two ends of a battery). This is called a proton motive force, and without it, electrons will not be able to move along the second “uphill” pathway. Once the proton motive force is produced from the downhill pathway, electrons can travel along the uphill pathway until they are accepted by the coenzyme NAD, which then forms NADH with hydrogen. NADH can then be used with carbon dioxide to produce sugar in the Calvin cycle.
What about eating electricity??
To test whether A. ferrooxidans can survive on electricity alone, without any iron, the scientists put the bacteria into a solution with a 0.4 V electrode, dissolved oxygen, and dissolved carbon dioxide (don’t worry about why just yet). Without any bacteria there was no electric current, but after adding the bacteria, they observed a current flowing from the electrode into the solution. They also found that the current increased with the number of bacteria attached to the surface of the electrode. This indicated that the [itg-tooltip tooltip-content=”<p>This means that electrons flow from an electrode to ions in a solution.</p>”]cathodic[/itg-tooltip] current resulted from the metabolic activity of the bacteria.
So, now they needed to figure out what happens to the electrons once they get inside the cell.
The electron transfer chain
The research team suspected that [itg-tooltip tooltip-content=”<p><strong>Heme proteins </strong>are proteins with iron in the middle. They function in many capacities, including electron transfer.</p>”]heme proteins[/itg-tooltip] would be involved in the electron transport chain. To test this hypothesis they took advantage of two pretty cool facts. (1) The ability of heme proteins to transfer electrons—their redox activity—can be blocked if they’re bound by carbon monoxide (CO). And (2), light can force CO to separate from heme proteins. When they treated the setup with CO, it did block the current flow from the electrode, and when they shined light on it, the current was restored.
So, they were on the right track. To figure out which heme protein is involved, they did some fancy tricks to see which wavelengths of light caused the current to increase the most. After finding them (430 nm, 545 nm, and 595 nm), they matched them to a known protein complex (the aa3 complex). So, now they knew that the downhill chain ends with an aa3 complex. If you skipped the cute figure above, take a look now. You’ll notice that when electrons are stolen from iron, the downhill chain also ends in an aa3 complex.
This led the researchers to test whether uphill transfer would also be similar and involve a bc1 complex and proton motive force. They were right. When they blocked the bc1 complex with the antibiotic Antimycin A, the current generation stopped, indicating that uphill transfer uses proton motive force and a bc1 complex, eventually leading to the formation of NADH.
What is all of this for?
The reason why electrons are passed along is so that eventually the bacteria can make sugars and grow (make more bacteria babies). To show that the bacteria actually grow when fed electricity, they counted the number of cells after 8 hours of access to the 0.4 V electrode and after 8 hours of access to a dead electrode without any voltage. They found that the number of bacteria cells increased only when they had access to the the electrical potential.
So, the team is now confident now that A. ferrooxidans can use electrical potential itself as an energy source to fuel growth even when no iron is available. Importantly, the electrical potential needed for this process is within the normal range of potential found at black smokers. The next steps are to figure out how A. ferrooxidans grabs electrons that are just sitting on an electrode with a voltage, and to search for other bacteria with the same ability that live near black smokers (A. ferrooxidans does not. It lives in caves). Finding electrolithoautotrophs at black smokers will go a long way to support the theory that not only do electrolithoautotrophic life and ecosystems exist, but that they might in fact be the first types of life to develop on Earth.