A research team led by Hokto Kazama at the RIKEN Center for Brain Science (CBS) in Japan has combined brain imaging and models of brain activity to explain how smells can be generalized into categories. The team examined a region of the fly brain that plays a central role in forming olfactory memories and discovered clustered representations of mixtures and groups of odors that are conserved across individual flies. This study, published in Neuron, explains how varying odors are perceived similarly in different individuals.
Recognition and generalization are essential processes that we often take for granted. Whether it’s recognizing a person’s accent or being able to categorize a never-before seen combination of foods as a type of pizza, somehow our brains do all the hard work in the background. In the case of smell, animals use their sense of smell to recognize food sources, predators, potential mates, and family. An odor is generally a mixture of multiple volatile molecules, yet animals do not recognize each molecule one by one, but rather the entire mixture as a single smell. Consider the citrus odor. Even though oranges, lemons, grapefruits, and yuzu all have different compositions of odor molecules, they can all be recognized as smelling “citrusy”.
To date, how the brain generates representations of unitary odor objects has not been well understood. Luckily, the ability to recognize and generalize odors can be accomplished by the common fruit fly, which has a very well mapped out olfactory system with a set number of neurons. The CBS research team focused on this system, particularly on the differences between two brain regions in the sensory pathway.
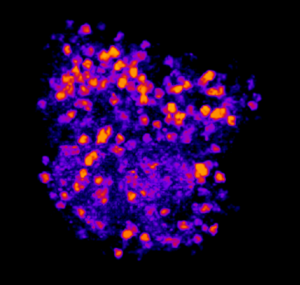
Calcium imaging captures the response to an odor from all 2000 neurons in the fruit fly mushroom body.
In the fly brain, odor information travels from the primary olfactory center, called the antennal lobe, to the secondary center, called the mushroom body. For their experiment to work, the team needed to simultaneously measure responses from many more neurons than has been done in the past. “Characterizing the activity of all 2,000 cells of the mushroom body at once was the biggest technical hurdle,” explains Kazama. “Previous studies have only recorded from less than 5% of them, which was not enough for our purposes.” They overcame this problem by developing an algorithm that automatically locates and tracks all the cells over an hour of recording.
They presented flies with 15 different odors individually as well as in mixtures and recorded neuronal responses by imaging the calcium released when the neurons were active. They found that clusters of neurons in the mushroom bodies responded selectively to individual odors, mixtures, or groups of odors. In contrast, neurons in the antennal lobe responded much less selectively. This tells that the mushroom bodies integrate input from the antennal lobe in some way and create distinct odor representations.
By using a mathematical model of neural information processing, the team was able to reproduce the results obtained from the real fly brains. Furthermore, they discovered that neural expressions of unitary odors in the mushroom bodies were similar among different individual flies, which can explain why odors are similarly recognized in different individuals. “The most surprising finding was that the same computation in the olfactory circuit can generate unitary representations for individual odors as well as groups and mixtures of odors,” says Kazama.
“Because the basic wiring pattern of the olfactory circuit is highly conserved across phyla, we believe that the type of computations we have discovered here may also be found in other more complex animals, such as humans,” Kazama says. “We are curious to know if these odor object representations remain stable or flexibly change over time as animals experience various odors in their environment.” ✅
Recognition and generalization are essential processes that we often take for granted. Whether it’s recognizing a person’s accent or being able to categorize a never-before seen combination of foods as a type of pizza, somehow our brains do all the hard work in the background. In the case of smell, animals use their sense of smell to recognize food sources, predators, potential mates, and family. An odor is generally a mixture of multiple volatile molecules, yet animals do not recognize each molecule one by one, but rather the entire mixture as a single smell. Consider the citrus odor. Even though oranges, lemons, grapefruits, and yuzu all have different compositions of odor molecules, they can all be recognized as smelling “citrusy”.
To date, how the brain generates representations of unitary odor objects has not been well understood. Luckily, the ability to recognize and generalize odors can be accomplished by the common fruit fly, which has a very well mapped out olfactory system with a set number of neurons. The CBS research team focused on this system, particularly on the differences between two brain regions in the sensory pathway.
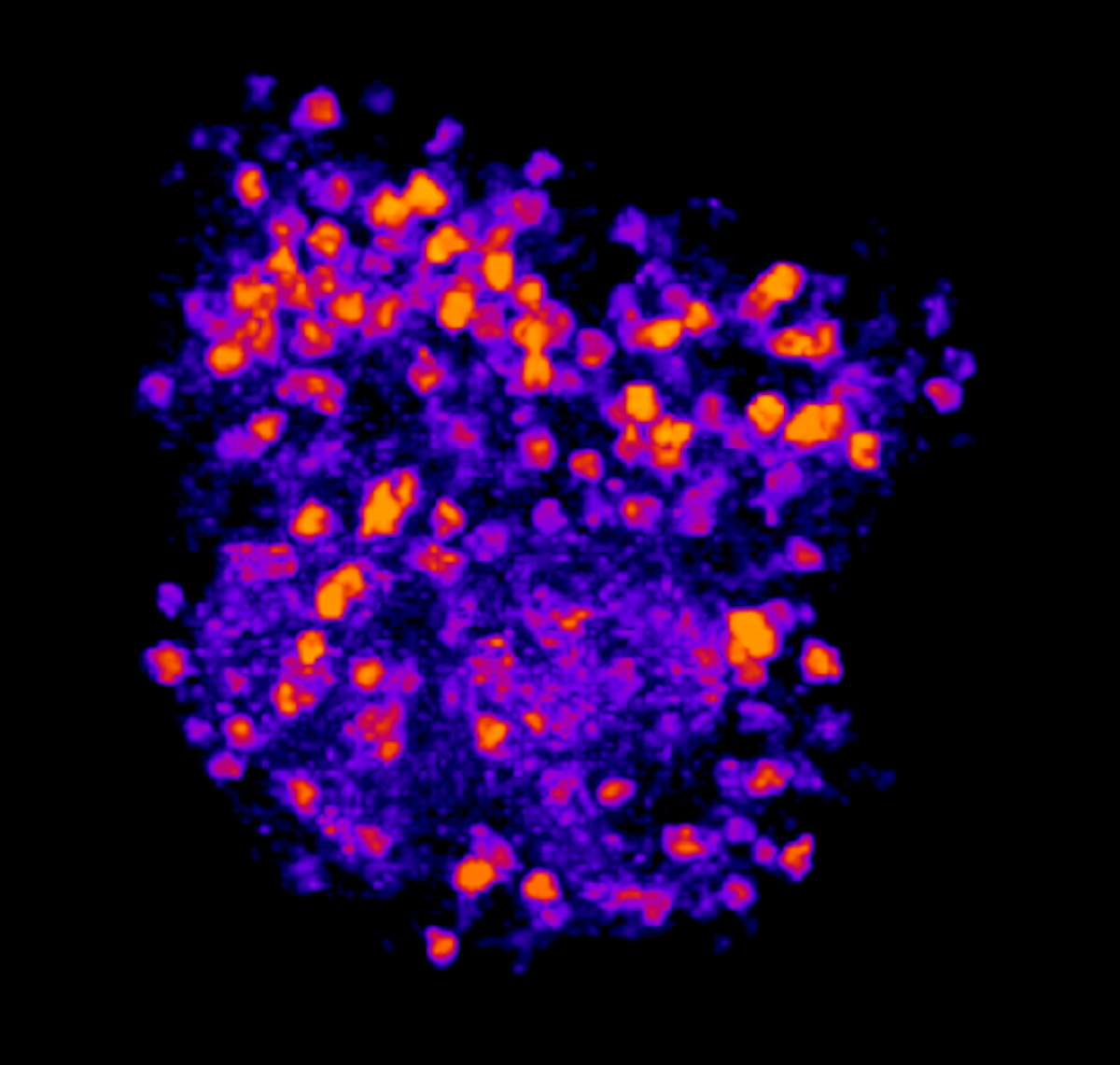
Calcium imaging captures the response to an odor from all 2000 neurons in the fruit fly mushroom body.
In the fly brain, odor information travels from the primary olfactory center, called the antennal lobe, to the secondary center, called the mushroom body. For their experiment to work, the team needed to simultaneously measure responses from many more neurons than has been done in the past. “Characterizing the activity of all 2,000 cells of the mushroom body at once was the biggest technical hurdle,” explains Kazama. “Previous studies have only recorded from less than 5% of them, which was not enough for our purposes.” They overcame this problem by developing an algorithm that automatically locates and tracks all the cells over an hour of recording.
They presented flies with 15 different odors individually as well as in mixtures and recorded neuronal responses by imaging the calcium released when the neurons were active. They found that clusters of neurons in the mushroom bodies responded selectively to individual odors, mixtures, or groups of odors. In contrast, neurons in the antennal lobe responded much less selectively. This tells that the mushroom bodies integrate input from the antennal lobe in some way and create distinct odor representations.
By using a mathematical model of neural information processing, the team was able to reproduce the results obtained from the real fly brains. Furthermore, they discovered that neural expressions of unitary odors in the mushroom bodies were similar among different individual flies, which can explain why odors are similarly recognized in different individuals. “The most surprising finding was that the same computation in the olfactory circuit can generate unitary representations for individual odors as well as groups and mixtures of odors,” says Kazama.
“Because the basic wiring pattern of the olfactory circuit is highly conserved across phyla, we believe that the type of computations we have discovered here may also be found in other more complex animals, such as humans,” Kazama says. “We are curious to know if these odor object representations remain stable or flexibly change over time as animals experience various odors in their environment.” ✅
Further reading
Endo et al. (2020) Synthesis of conserved odor object representations in a random, divergent-convergent network. Neuron. doi: 10.1016/j.neuron.2020.07.029
Further reading
Endo et al. (2020) Synthesis of conserved odor object representations in a random, divergent-convergent network. Neuron. doi: 10.1016/j.neuron.2020.07.029